Silicon Carbide -- a Little-Known Mineral Changing Our World in Big Ways
by Andy Thompson, MSDC Secretary
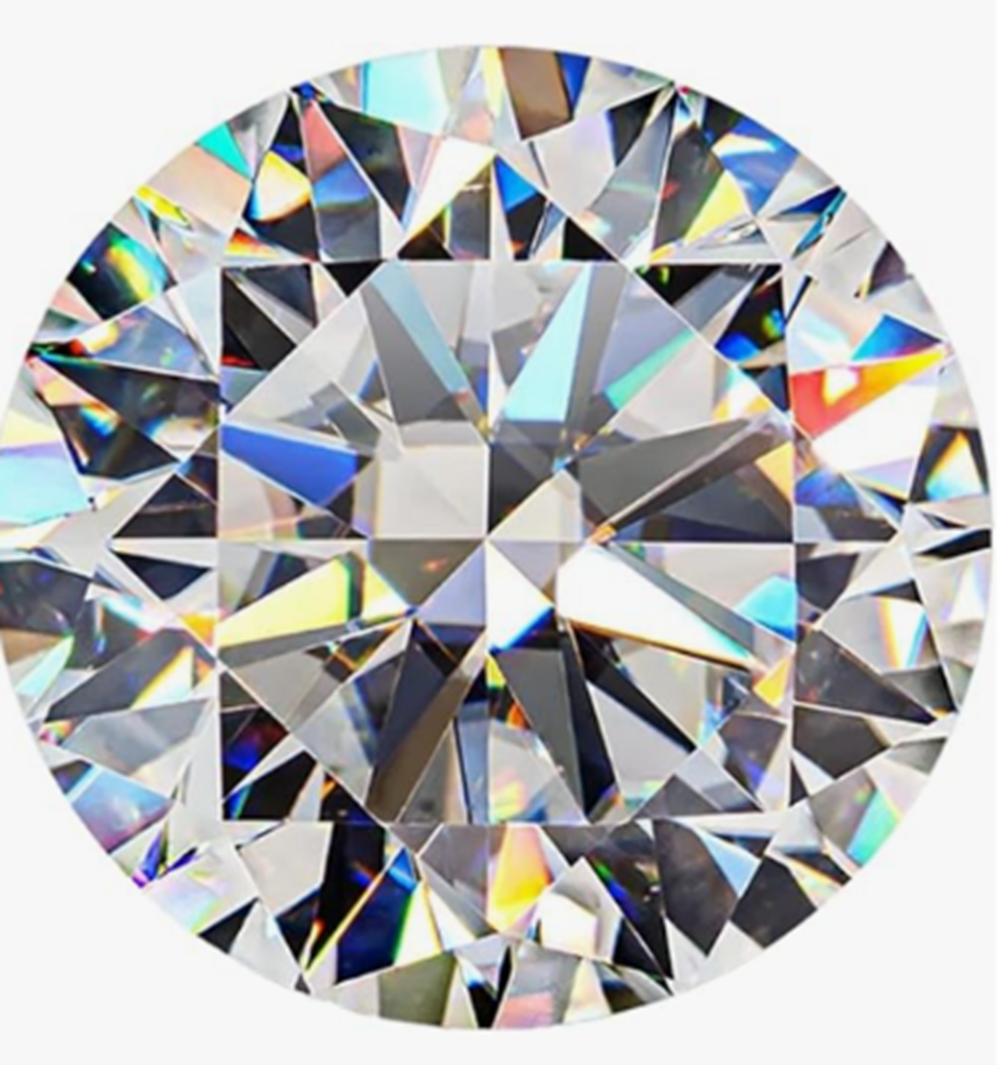
The purpose of this article is to tell a bit of the little-known story of the mineral moissanite also known as silicon carbide (SiC) and carborundum. For the last three decades this mineral has been playing a central role in changing the ways we are lighting our cities and empowering our electrical grids tomorrow.
Moissanite, in its natural form, is a rare and microscopic mineral. In that form, it seems to be a safe bet that few collectors have specimens on their shelves. Discovering it in nature is extremely rare because it is found almost exclusively in meteorites and then only in microscopic size. Astrophysicists, however, assure us it is abundant as star dust in the formation of certain nova. But aside from its sparse presence in meteorites, in its natural form, SiC is little known on planet Earth. For rare occurrences, search "moissanite" in mindat.org.
Because this natural variety of SiC is extremely rare, it seems to have not been widely discussed in the mineral community. Simply put, its molecule consists of five atoms – one central carbon and four silicon atoms that form very strong tetrahedral covalent bonds, as illustrated below.
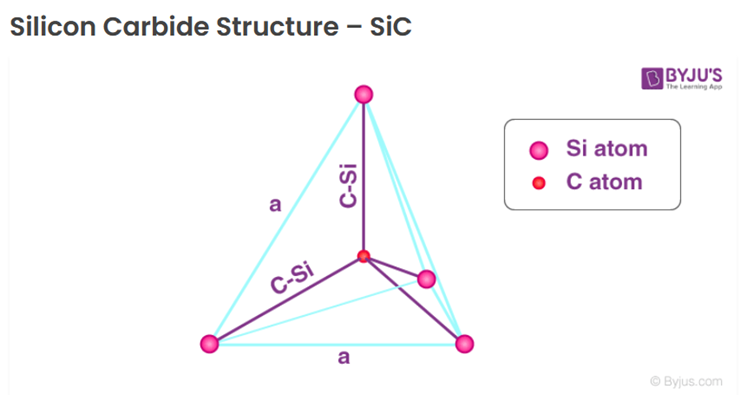
The covalent links mean the atoms share their electrons (two each) and that may help give SiC crystals “super powers” which recent developments and applications described below have been exploiting. By the time readers reach the end of this article, some may be surprised to discover: “Oh yes, I have heard about many of these tech developments.”
The History of Moissanite
This mineral has been referred to by three names, moissanite, silicon carbide (SiC) and carborundum, depending on its level of purity, its context, and use. The discovery of the natural mineral moissanite came about in 1893 when a French chemist, Henri Moissan, was searching for a mineral that could replace copper and be a more efficient conductor of electricity. That mission brought him to Canyon Diablo in Arizona where he was digging in a meteor crater, perhaps on the assumption that such impact craters, with their traumatic history, sometimes generate interesting mineral compounds. He was awarded a Nobel prize in 1906, but it was for isolating fluorine, not for discovering moissanite delivered by a large meteorite.
The vast majority of meteorites, but especially chondrites, are composed of silicate minerals, including minute grains of SiC dust, as well as ultramafic minerals including iron and magnesium. Initially, Henri Moissan believed he found a very tiny diamond crystal. Later he learned it was silicon carbide. A decade after his discovery geologists credited him by naming the molecule in his honor.
During that same time frame of the late 1800s and early 1900s, a start-up company in the northern Minnesota iron region began mining, smelting, and manufacturing a crude mix of iron and other minerals including silicon and carbon. This process produced an abundance of low-grade silicon carbide powder, also known as carborundum, which they marketed extensively as abrasives.
This powder was found to have extensive practical uses such as in wet sandpaper, sanding stones (pictured below), and other domestic and very low-tech industrial applications.
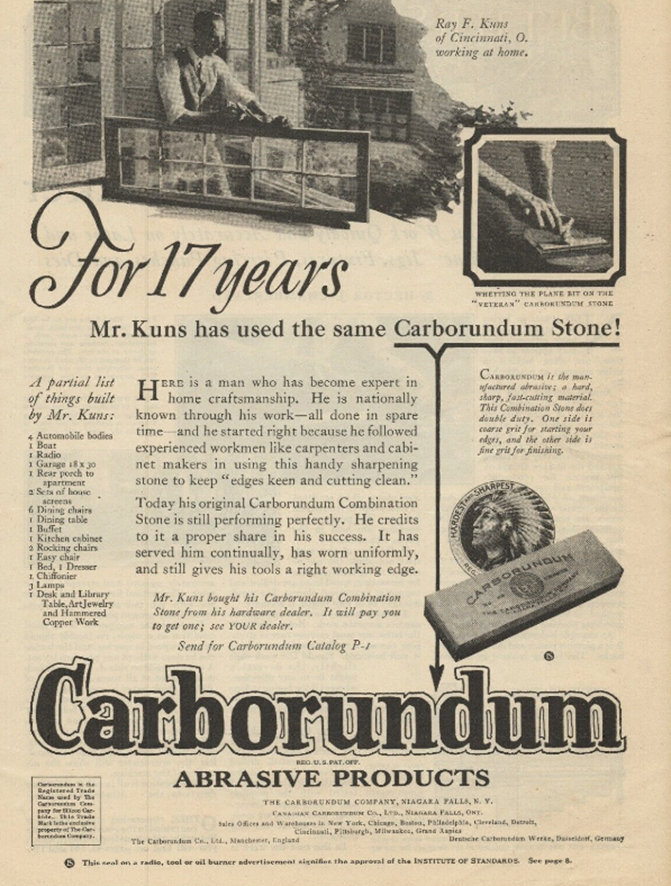
Today that company is known as the international giant, 3M, abbreviated from Minnesota Mining and Manufacturing.
Below is an image of a colorful iridescent mineral specimen of synthetic carborundum. These minerals are often the accidental byproduct of smelting a mélange of metals, such as iron or zinc with silicates, carbon, and flux materials in high temperature furnaces. These specimens qualify as prettyite, not leaverite, and are manufactured examples of SiC that might be found in a mineral collector’s showcase.
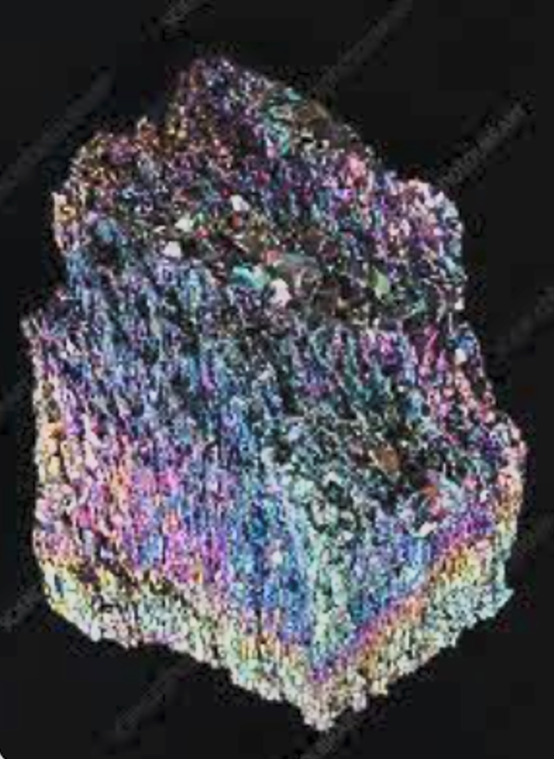
High Tech Forms of Synthetic Silicon Carbide Crystals
Fast forward about 70 years from the 1920s and we find materials scientists and researchers from the University of North Carolina in the 1990s striving to develop defect-free SiC crystals for transforming the semiconductor industry. Along the way they also made the Edison incandescent light bulb obsolete. That revered icon could understandably be called a “heat bulb” given that it literally generates more heat than light. In our energy-starved world, advancing beyond the Edison bulb has been crucial because lighting fixtures used about 15 percent of all our available electrical energy in the U.S. in 2022. Before the advent of LEDs, the wasteful expenditure of electrical energy spent on lighting was much higher.
That small group of UNC researchers helped transform the lighting industry by creating blue LEDs which, for the first time, used purified tiny silicon carbide chips. These blue LEDs first appeared commercially in Volkswagen auto dashboards. But when combined with already available red and green LEDs, the trio generated the energy efficient white lights now found throughout many of our homes and highways.
That company, Cree Research, now called Wolfspeed, also provided SiC semiconductors, meaning the chips can act as a conductor or insulator of electrical current, and were free of inclusions, flaws, or gaps in their crystal structure. This property made them suitable for advanced electrical applications such as metal-oxide semiconductor field-effect transistors (MOSFETS) that are used in switching or amplifying signals and especially useful in extreme temperature situations on Earth and in outer space.
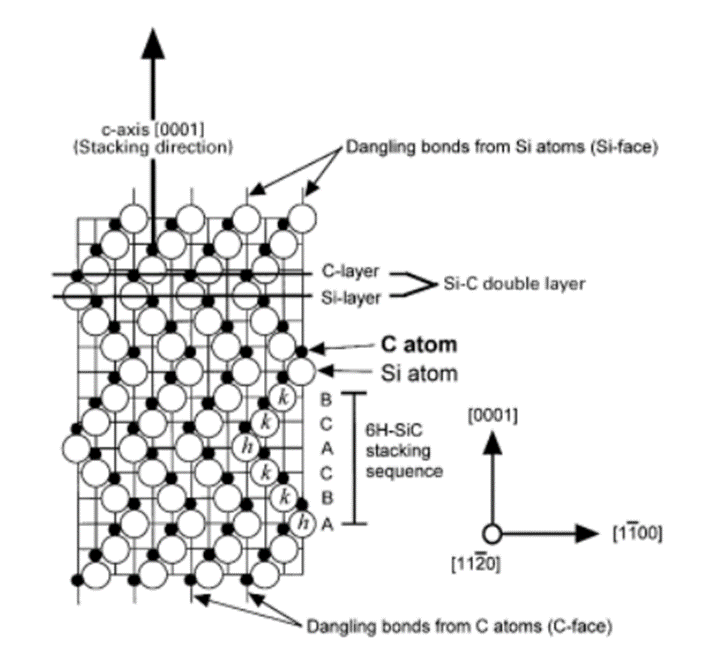
Cree introduced its SiC-based LEDs just one year behind competitor Nichia Corporation whose dedicated, then-relatively unknown, top researcher developed the world’s first blue LED. Shuji Nakamura, pioneered the use of gallium nitride to make blue LEDs for which he received the Nobel prize in physics in 2014.
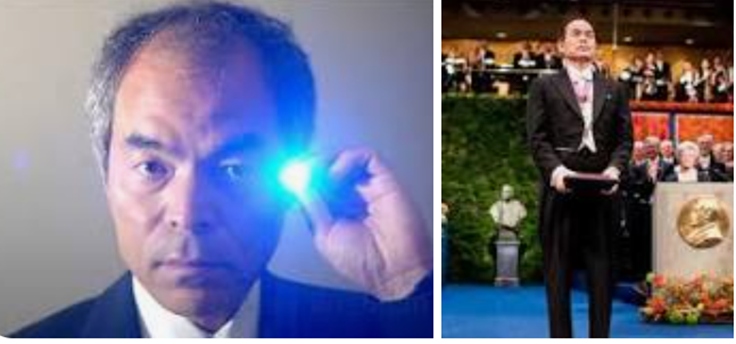
It took Cree decades of intense trial and error with silicon carbide and gallium nitride to purify their precision crystal lattice structures and make them free of any defects, as required for use in today’s high-tech applications.
Looking Back and Forward with SiC
The path to developing the high-tech “super powers” of pure SiC minerals began with geologist Henri Moissan’s simple discovery of SiC. During the early 1900s, given SiC’s near-diamond hardness, about 9.25 to 9.5 on Mohs’ scale (versus 10 for diamonds), the manufacturers used it primarily as an abrasive. A century later, today’s researchers are tapping its capability to perform as a semi-conductor, as an on/off switch, in extreme temperatures and high voltage conditions, due to the strength of its molecular bonds.
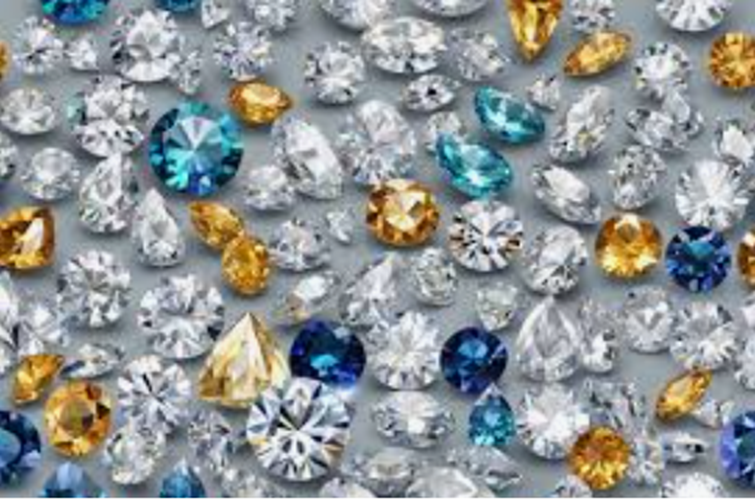
Readers may well ask: how does a moissanite gemstone, such as is pictured at the beginning of this article and above, fit in with the above tech developments? In short, the same company, Cree Research, uses the same process – vapor deposition – to create both moissanite gemstones and semi-conductors. The synthetic gemstones were developed by Cree in the 1990s and produced by its spin-off company initially called C3, later renamed Charles and Colvard. The synthetic gems have been marketed as less-expensive substitutes for diamonds. Because of their brilliance, jewelers initially had difficulty distinguishing them from diamonds and some said moissanite was superior in dispersing light.
The SiC moissanite gemstones are produced in high temperature ovens (over 4,000 degrees F) by super heating an open container of SiC powder which becomes a gas and is then deposited on a receptive surface. In contrast, the SiC used in producing semiconductors demands far greater purity. Its deposition process results in a cylindrical boule, shown below.
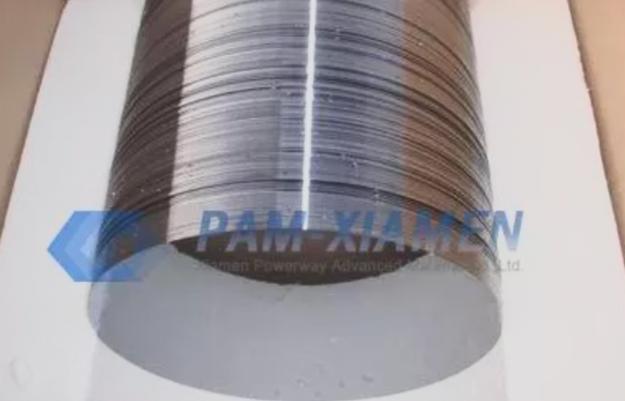
The boule is then sliced into extremely thin Wolfspeed disks, shown below, which are then imprinted with microscopic circuits. The disc is subsequently cut into many thousands of tiny chips for diverse applications in high tech circuitry.
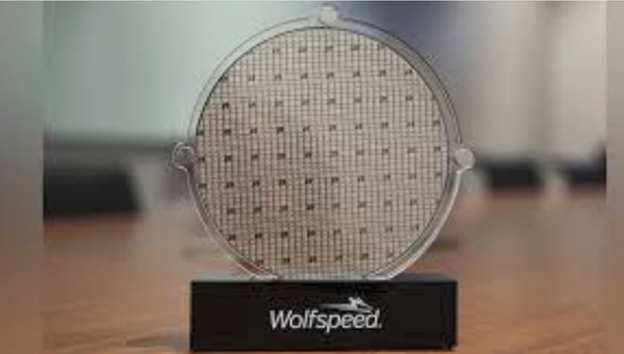
Silicon Carbide’s New Use in Hot Rocks
Briefly put, here’s the second of our two stories. Energy engineers have discovered a new use for SiC which promises to transform our ability to generate and store inexpensive electrical energy.
In 2023, as recently as mid-December, news agencies have featured stories of how several start-up companies discovered a way to convert wind and solar green energy into what journalists named “hot rocks” which then can serve as thermal batteries to store energy for considerable periods. The rocks’ heat may be stored for long periods and then converted to electrical energy days later, as needed, even when the green energy wind mills stop turning and the sun ceases to shine on solar collectors.
Here’s the basic idea. Imagine you are present a million years ago and you witness a smarter-than-average cave man or woman who one cold night gets the bright idea of taking one of the heated rocks bordering the clan’s fireplace and moving it closer to the family’s sleeping area. Capturing the rock’s heat helped provide the family members with a few hours of comfortable, warmer sleep and enhanced their quality of life. And therein we witness the discovery of an early thermal battery.
Fast forward to more recent times. Our energy-starved nations are searching for ways to strengthen overstressed energy grids which are increasingly challenged to meet today’s demands to heat our homes, power our electric vehicles, and run our factories. Burning fossil fuels, important as this ancient process has been, is no longer up to today’s demanding energy requirements.
Is there a way our clever ancestor’s innovative use of the clan’s hot-rock-technology can help with today’s expanding energy challenges? I came across the stories of two small start-up companies which are using “hot rocks” as innovative thermal batteries.
Antora Energy of Fresno, California, does exactly that by putting graphite rocks into a super-heated steel box, shown below. Their graphite rocks retain the heat energy for days and are inexpensive. Moreover, the graphite is expected to remain structurally sound for many decades.
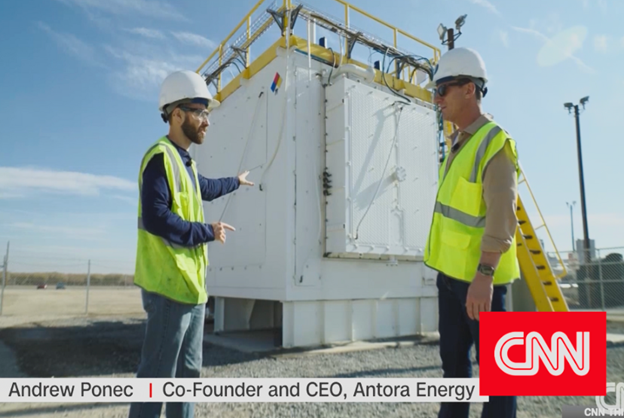
The Fresno, CA startup company has been supported, in part, by Bill Gates. Its young CEO, Andrew Ponec, shown above on the left and in front of their steel oven, explains its technology to interviewer Bill Weir.
“There’s not much more here than a steel box, with insulation inside of that and carbon blocks inside of that.” It’s simple and that’s the point. The graphite blocks are heated to 1,600 degrees Celsius and that heat can be retained for days and then converted into steam and then into electricity to supply domestic and commercial industry. The key development is that the super-heated rocks serve as thermal batteries which retain their energy far longer than our ancient ancestor’s cave rocks and perform better than other forms of large-scale stored energy.
If readers desire more information on Antora’s story, see the short video: Energy storage of the future? 'Hot rocks' in a box | CNN | wfmz.com
Silicon Carbide Now Enhances Hot Rock Technology
Rondo Energy is another California startup located in the Bay Area that addresses the issue of how to efficiently capture and store green energy when solar and wind are not available. Although similar to Antora’s heated-bricks technology, this method uses refractory bricks containing oxygen, silicon, aluminum – Earth’s most abundant minerals – and importantly, silicon carbide.
Rondo CEO John O’Donnell said “decarbonizing industrial heat is a trillion-dollar market requiring far more storage than the electric grid. The technology is here now. The demand is here now. This planned expansion means that the capacity is here now as well.”
SiC, with its super power to retain and evenly distribute very high temperatures, enhances this hot brick technology. And, unlike the semi-conductor industry, this hot-rock-thermal-battery technology comes without any need for 100% purity in its SiC crystals. It does not need to be inclusion-free. Nor is there any need for eliminating absolutely all defects in its molecular crystal structures.
If interested in Rondo’s approach, see: Rondo's brick battery factory set to become the largest in the world (smart-energy.com).
For more information on refractory bricks, see: Refractory Brick Vs Fire Brick: What's the Difference (saint-gobain.com).
Conclusion
If any readers have made it to the end of this article on the little-known silicon carbide mineral, I want to congratulate you on your endurance and thank you for your interest. If you are already familiar with SiC and have any corrections or additions to the story as here told, your contributions are most welcomed. (thompson01@starpower.net